How it works, how it complements other energy, and how fast the market is maturing
CCEBA sat down with Blair Kendall, a long-time solar developer based in Durham, North Carolina, who is making a transition to geothermal. In this first of two installments we discussed how new technology is changing a power source that dates back to the Roman Empire.
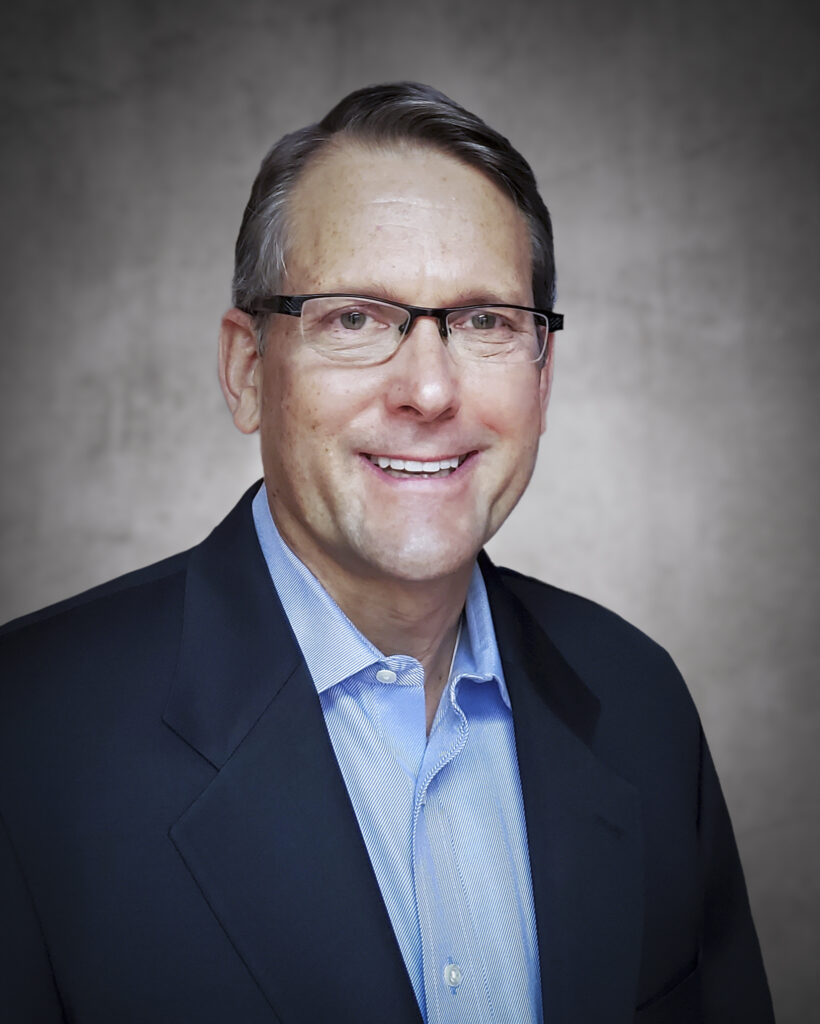
Q: What is Geothermal energy?
Geothermal energy, in its simplest form, means exploiting the heat under the surface of the earth for energy—heat (BTUs) or electric power (kWh). The core of the Earth is 7,200-9,000° F, and this heat emanates up to the surface. Heat is also generated by the decay of radioactive isotopes in the Earth’s crust. So, the deeper you go, the hotter it gets.
Geothermal energy isn’t new. The Romans used natural hot water springs to heat their buildings and baths. And, on the power side, the first geothermal power plant was built in Larderello, Italy in 1904.
Geothermal for heat, or thermal energy, is a relatively mature technology with residential, commercial and industrial applications. In each of these cases, hot water is extracted from ground, passed through a heat exchanger to capture the BTUs, and then pumped back into the ground through an injection well.
Since CCEBA members develop power plants, I am going to focus on the power side of the equation. With electric generation, the wells are much deeper (6,000 to 25,000 feet), and the water is much hotter (225° F to 700° F). When looking at geothermal you need to break it into the surface facilities or power plant type, and then the subsurface wellbore and reservoir component.
Starting on the surface. Traditional geothermal, or hydrothermal, which has been around since the 1970s, uses high temperature water from naturally occurring, deep subsurface reservoirs to directly extract steam from the reservoir (Dry Steam) or bring up hot water which is used to create steam (Flash Steam) to drive a turbine and generate power. For these power plant types the temperature of the reservoir water is ~ 300-700° F.
More recently, geothermal developers are utilizing binary cycle power plants, also called Organic Rankine Cycle technology (ORC), to generate power at temperatures as low as 200° F. With ORC plants, the hot water is passed through a heat exchanger. The primary loop runs the hot brine from the subsurface through a heat exchanger to heat the working fluid in the secondary loop. The working fluid, which has a lower boiling point than the water, generates steam to power the turbines. ORC engines are less efficient than the traditional hydrothermal plant but have lower operating costs, less environmental impact, and can operate at lower temperatures. With all three types of power plants, the water is then cooled and reinjected back into the subsurface reservoir through an injection well.
Q: What is the state of the geothermal market?
The U.S. has almost 4 gigawatts (GW) of operating geothermal power. Most of this capacity was developed in the 1970s and early 1980s in northern California and western Nevada. The largest geothermal installation is The Geysers, a complex of 22 geothermal power stations located in California. When commissioned in 1960, this was the first geothermal area to be exploited for power generation in the U.S. It has since been developed into the largest geothermal field in the world, with a nameplate capacity of 1,517 megawatts (MW).
This traditional geothermal, or hydrothermal power is limited to only a few areas where all the necessary factors align, including subsurface heat, permeable rock and abundant water. Because of the constrained geography of this resource, traditional geothermal hasn’t taken off like wind and solar. Quite frankly, interest in traditional geothermal has waned over the past three decades.
Q: Why is everyone suddenly talking about geothermal again?
In the past few years, the mainstream energy and business press have been buzzing about “next-gen geothermal.” A myriad of new technologies, a growing ecosystem of start-ups, new capital investment, and a strong policy push are driving a resurgence of interest in new geothermal energy.
There is a convergence of new technologies which are being applied to new geothermal, enabling and accelerating its development. In the oil and gas (O&G) industry, the last few decades have seen major advances in drilling technology, including horizontal drilling, fracking, and subsurface analysis technologies. On the geothermal side, Ormat, the leading global geothermal developer, popularized the application of the Organic Rankine Cycle (ORC) technology for utility-scale geothermal generation. ORC engines aren’t new, but in the past they were mainly used for capturing industrial waste heat. Lastly, the rise of cloud computing and AI has facilitated sophisticated modeling of the Earth’s subsurface, better enabling detection and use of this underground heat energy.
Q: So, what exactly is “next-gen geothermal”?
Traditional geothermal needs three factors to line up: High heat close to the surface, permeability of the rock, and abundant water. With advanced drilling technologies, and in some cases, fracking, you can now cost-effectively drill deeper to find this underground heat and can create the permeable fracture system necessary to engineer an artificial reservoir. All of this has opened many more regions in the U.S. and world for geothermal development
There are a few different next-gen technologies in play. The two getting the most attention are Enhanced (or Engineered) Geothermal Systems (EGS) and Advanced Geothermal Systems (AGS). With EGS, the vertical production and injection wells have long horizontal wellbores, and they frack impermeable hot rock to create long cracks in the rock connecting these parallel wellbores. The water is pumped through this man-made reservoir where it heats up and is then brought to the surface to power the turbine. Depending on the temperature, the plant may be a Flash plant or an ORC engine. While EGS has been around since the 1970s, these early projects demonstrated little commercial potential because many of the underlying technologies we are using now didn’t exist.
The leader in EGS right now is Fervo. In the past 24 months, Fervo successfully completed its first pilot near the Blue Mountain powerplant in Nevada where they drilled, completed, and tested a three MW system with Google. The produced water is being piped to an existing geothermal power plant operated by Cyrq under an existing power purchase agreement (PPA) with Nevada Energy. Fervo also recently began drilling its first utility scale commercial project in Utah with Southern California Edison as the offtaker. In January, Fervo raised $244 million to scale up the company.
AGS, also called closed-loop geothermal, drills a radiator-type configuration of wellbores in the subsurface and then runs the water through this system to pick up the subsurface heat. Like EGS, AGS depends on conductive heat transfer to recharge the energy captured from the rock. Here the leading companies are Eavor and Greenfire Energy. Eavor completed two successful pilot projects and recently broke ground on a 200 MW project in Germany. Greenfire demonstrated the viability of its technology with the Coso project in California and is now evaluating several utility-scale, commercial projects in Kenya, Taiwan, and the U.S.
Another next-gen pioneer is Sage Geosystems, which is focused primarily on energy storage. For me, I think their storage technology is the break-out innovation. In its simplest form, it is like pumped hydro turned upside down, or pumped hydro in the earth.
I am not doing all this justice—I could go on for hours about all the innovative start-ups in the next-gen space, such as Quaise and its plasma drilling technology, or GTI and its Hot Sedimentary Aquifer technology. Check those out if you have the time.
Thank you for a great summary of the hidder power!